Optimising offshore wind
Researchers from the Offshore Renewable Energy Catapult and Northumbria University, UK, wind up the next generation of smart offshore windfarms.
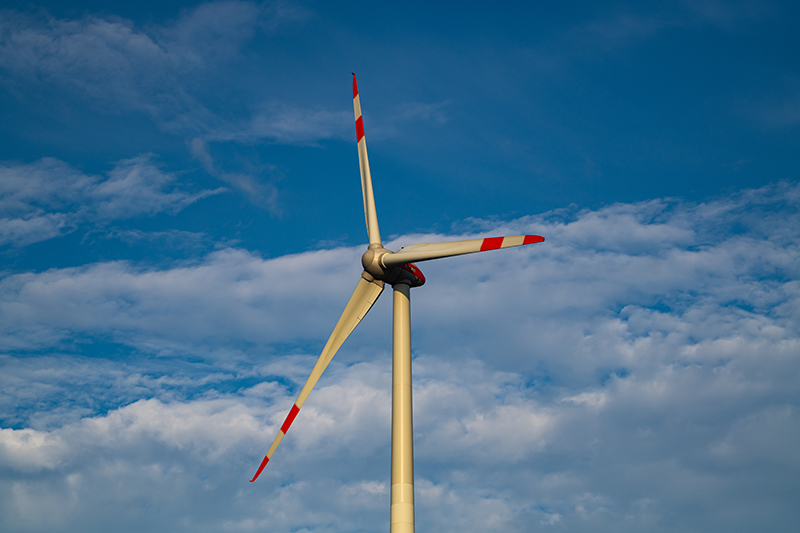
Offshore wind is increasingly the preferred solution for the UK’s short- and long-term energy strategy as an efficient renewable energy source. It has gained substantial traction compared to onshore wind farms, owing to its capacity for large-scale renewable energy generation and its ability to access stronger and more consistent winds.
There are plans to increase the installed offshore wind capacity from about 10GW in 2020 to 50GW by 2030, delivering approximately 175TWh of electricity – almost half of the current annual electrical energy consumption.
The core of the electric system is the offshore wind turbine equipped with large rotor blades that capture the kinetic energy of the wind and convert it into mechanical energy, then convert it into electrical energy through the generator.
The turbines are installed in bodies of water, typically in oceans or seas, to harness the wind’s energy. Depending on the water depth and other site-specific factors, they are mounted on fixed foundations (monopiles, jackets, gravity-based structures) or floating platforms.
Their size is among the largest in the world. They are larger and more robust than onshore turbines to withstand the harsh marine environment. The rotor diameters range from 100m to more than 200m, and generate capacities of several megawatts.
The electric system encompasses various components that allow for the efficient generation, transmission and integration of electricity produced by offshore wind turbines.
The output is typically in the form of variable-frequency alternating current (AC). Power converters change the variable-frequency AC signal into a more stable power signal at a standardised frequency suitable for power transmission.
The turbines are connected to an offshore or substation platform. This platform collects the electricity generated by multiple turbines and houses the necessary equipment for voltage transformation, frequency conversion and data communication. The collected electricity is then transmitted to the shore through underwater power cables.
Offshore wind farms need to be seamlessly integrated into the onshore power grid. This requires cooperation among different sectors, including energy generators, utilities and grid operators, to ensure grid stability by managing fluctuations in electricity supply and demand.
Accessing and maintaining offshore wind turbines can be challenging due to their remote location. So advanced monitoring systems and maintenance strategies are employed to ensure optimal performance and longevity.
Research and development is focused on improving turbine efficiency, reducing costs and enhancing reliability. Innovations in blade design, development of floating turbine technology and remote monitoring capabilities have been driving the sector.
Did you know?
In the first quarter of 2023, renewable sources (wind, solar, biomass and hydro) accounted for 42% of the UK’s electricity, with wind power alone providing nearly a third (32.4%), according to power generation company Drax. Meanwhile, gas-fired power generated 31.7% of the overall electricity.Leading-edge erosion
Offshore wind turbines are generally designed for 20-25 years of operation service. Their performance significantly depends on the aerodynamic properties of the turbine blades. However, a significant challenge is controlling and mitigating leading-edge erosion damage caused by repeated bombardment on the turbine blade, primarily from raindrops and particulate matters like hail, ice, salt and other forces in highly corrosive environments.
As the particles impact the blade’s leading edge, they cause damage and remove material from the surface, resulting in a roughness profile that diminishes the aerodynamic performance. This can result in reduced turbine efficiency and costly repairs, leading to power generation losses and reduced availability.
Frequent inspection of the blade is therefore needed to spot leading-edge erosion. One of the popular methods is to use drones for close-range inspection of the blade surface by acquiring images or videos. The accumulated visual data can lead to classifiers on databases for assessing damage on turbine blades, and provide corresponding suggestions for repair or decommission.
To maximise the operational lifespan of the turbine, decrease inspection frequency, and achieve cost-effective operations and maintenance, the current trend is to prioritise the material and structural integrity both for existing and upcoming offshore wind turbines.
Did you know?
In the first three months of 2023, Britain’s growing number of wind turbines generated more electricity than gas-fired power stations for the first time.
Wind turbines generated 24TWh of electricity in the first three months of 2023 and the output was 3% higher than the same quarter last year, according to figures from Drax.
6G in the mix
A potential, future, smart inspection technology are digital sensors inside the turbine blades for continuous damage monitoring, especially on the leading-edge area. Using advanced artificial intelligence (AI) technology, inspections can be recorded and instantly analysed through a scalable cloud system that conducts consistent data acquisition and high-quality predictive analysis.
The next six-generation (6G) mobile communication technology can establish a new standard to fulfil the high requirements for more intelligent networks, extreme network communication speeds and support a vast number of connected devices. It can be used for continuous onsite turbine blade inspection.
While 6G technology is currently in its early stages of R&D (commercial deployment is anticipated later in the decade), it holds the potential to enhance future wind turbine systems.
The ultra-high-speed data transfer and low-latency capabilities can significantly improve the communication between wind turbines in a wind farm. This enhanced communication can optimise turbine performance, enable real-time monitoring and improve coordination in response to changing wind conditions.
Wind turbine operators can remotely monitor and control turbines with greater efficiency and responsiveness using 6G technology. The combination of low latency and high bandwidth with AI-driven algorithms can enhance automation within wind turbine systems.
AI could also be used for predictive maintenance, fault detection and power-output optimisation, leading to higher efficiency and reduced downtime. Also, real-time monitoring and analysis can help detect and prevent potential risks or security breaches.
6G can ultimately facilitate the seamless integration of wind turbines with smart grids, enabling real-time data exchange between energy producers, consumers and grid operators, leading to a more efficient and sustainable energy supply.
Winding down
By 2025, the UK Government and renewable energy industries must prioritise the decommissioning of offshore wind turbines as they approach the end of their operational lifespan.
Within each early-model turbine, thousands of components and parts have undergone wear, replacements and repairs without clear estimates of their installation timeframe, and many of these components are now approaching the end of their expected lifespan.
Approximately 300-1,600 early-model offshore wind turbines must be decommissioned by 2025 and 2030, respectively.
This situation underscores the necessity for immediate action to make the best use of the remaining time before turbines installed in the 1990s and early 2000s become unsafe and non-functional by 2025. Failure to act can lead to safety issues, substantial costs and the permanent loss of the expertise needed for a safe and successful decommissioning process.
Offshore wind turbine technology continues to evolve, with ongoing R&D aimed at increasing efficiency, reducing costs and enhancing the integration of renewable energy into the global power supply. As technology advances, offshore wind power is expected to play an increasingly significant role in the transition to a more sustainable energy future.
Integrating hydrogen
Integrating wind electricity and hydrogen creates a symbiotic relationship that addresses the challenges of wind-power intermittency and provides a scalable energy-storage solution.
Power-to-Hydrogen (P2H) is a process that involves using excess wind electricity to produce hydrogen through electrolysis. During periods of high wind energy generation, when electricity demand is low, surplus power can be diverted to electrolysers. Electrolysers split water into hydrogen and oxygen, with hydrogen being the valuable energy carrier.
This hydrogen can be stored in tanks or underground facilities until needed. When electricity demand exceeds the available wind power supply, the stored hydrogen can be converted back into electricity. This provides a flexible energy supply that can help balance the grid and meet electricity demand when wind power output is low.
Hydrogen produced from wind electricity (green hydrogen) is a clean and low-carbon energy source. It can be used in various sectors to replace fossil fuels and reduce greenhouse gas emissions. Green hydrogen can be used in fuel cell vehicles or blended with natural gas in existing gas pipelines for heating purposes.