MXenes making their mark
Graphene dominates the 2D materials circuit, however Professor Yury Gogotsi and Armin Vahid Mohammadi at Drexel University, USA, suggest MXenes are ready to make their move.
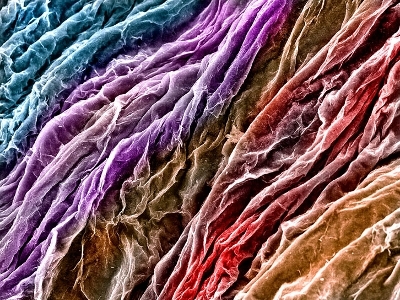
Two-dimensional (2D) nanomaterials are set to affect many aspects of our lives by changing human-to-human and human-to-device interactions. Their potential comes from the materials' unusual properties, high-aspect ratio and unique 2D structure, essentially becoming nanoscale Lego bricks.
Among the many 2D materials, including graphene and phosphorene, MXenes, which are 2D transition metal carbides and nitrides, have shown significant promise due to their variety of structures and chemical compositions. They have outperformed other 2D materials in many applications, including energy storage, structural alloys and electronics due to their metallic electrical conductivity, dispersibility in water (like clay minerals) and simple processing into various forms and shapes such as films, powders, aerogels, printed patterns and fibres.
Scaling up
Discovered in 2011 at Drexel University, USA, MXenes are generally produced from bulk layered carbide and nitride precursors – known as MAX phases – through an aqueous or molten salt chemical process. This results in powders (multilayer particles) or single-layer flakes of MXenes with a nanometre to sub-nanometre thickness. Because of this wet chemical synthesis from powdered ceramic precursors, MXenes can be produced in large quantities and scaled-up with few difficulties.
Their structure comprises one to four carbon and/or nitrogen layers (X sites) sandwiched between two to five transition metal layers (M sites, such as titanium, vanadium, niobium, etc.) The schematic on the page opposite demonstrates MXenes' known structures. The M sites can host more than one transition metal in the form of solid solutions, e.g. (Ti,Nb)₂C or an ordered sandwich-like structure e.g. Mo₂TiC₂. Therefore, unlike graphene and other 2D materials that only exist in one or a few chemical compositions, MXenes can be made in many different compositions and structures. For instance, the first discovered and still the most studied MXene, Ti₃C₂, is formed of abundant elements and is biocompatible and non-toxic, making it attractive for many applications.
A large and happy family
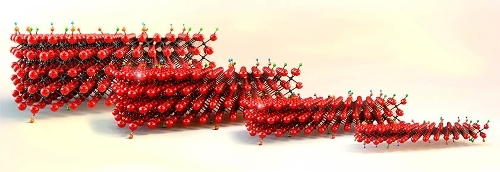
There are more than 30 different stoichiometric compositions that have been experimentally made, and more than 100 have been theoretically predicted. MXenes are therefore considered the largest family of 2D materials, affirmed perhaps by their ability to form solid solutions on M and X sites, as well as a variety of surface terminations (oxygen, hydroxide, fluorine, sulphur, chlorine, bromine and tellurium have been shown).
In 2013, MXenes’ ability to host a variety of positively charged ions (cations) was demonstrated – a key milestone that means the capacitive charge storage performance of MXenes surpasses carbon materials, capturing the attention of energy storage researchers.
Initially, the only effective chemical for synthesising MXenes from layered MAX phase precursors was hydrofluoric acid (HF). In 2014, however, it was shown that that HF could be replaced by a milder and less harmful solution containing hydrochloric acid and fluoride salts such as lithium fluoride. While being less hazardous, this new process revealed the clay-like rheological behavior of MXenes so that they could be turned into films and fibres for different applications. Later on, molten salt and electrochemical etching synthesis methods of creating MXenes were also published.
Another key breakthrough came in 2016, when titanium carbide MXene films revolutionised the field of electromagnetic interference (EMI) shielding. The films presented the highest effective EMI shielding among all known synthetic materials, including thin metal plates that are widely used in this industry.
More recently, titanium carbonitride, another MXene composition, was shown to have an anomalously high absorbance of electromagnetic waves, even when compared with the titanium carbide MXene. It means this property, which arises from free electrons of transition metal atoms and their layered structure, could result in a new generation of lightweight sub-micrometre coatings and micrometre-thin freestanding films. This would protect electronic devices from electromagnetic noise, safeguard credit cards and cell phones, and facilitate the development of miniaturised electronics used in Internet of Things (IoT) devices.
MXenes are highly conductive due to the presence of metal carbide or nitride core layers in their structure, and their electrical conductivity in multilayer films surpasses reduced graphene oxide reaching beyond 20,000Scm-1. Furthermore, due to their strong ionic transport properties and low energy barrier for ion diffusion, they can store energy at a much faster rate than conventional electrode materials used in batteries and supercapacitors. This enables super-fast charge storage technologies and devices with millisecond charging times, capable of AC–DC current conversion.
Conductive MXene films can also be a substitute for other components inside battery cells, such as aluminium and copper current collectors, resulting in significant weight reduction. Moreover, MXene films and coatings can change their colour and transparency while being charged because of the electrochromic effect in these materials. By using this property, energy-storing, colour-shifting windows and coatings can be fabricated. MXenes’ excellent conductivity, their facile processing, and ability to form colloidal solutions in water means the materials can be used in conductive textile and fibre technologies. For example, they can be coated onto different types of fabrics, or directly processed into additive-free fibres.
Beyond inks
The hydrophilic nature and negative surface charge of MXenes create stable colloidal solutions and inks that are ideal for writing, printing, stamping or painting, with no need for additives. The addition of additives to formulate inks made of carbons or metals is a major drawback since polymeric additives negatively affect the electronic and mechanical properties of the printed patterns. MXene inks can also be used to print antennas and RFID tags for 5G communications and IoT devices, as well as for miniaturised sensors, batteries and supercapacitors used in flexible and wearable technologies.
Because of the covalent primary bonds between the transition metals and carbon or nitrogen atoms, MXenes are also among the strongest of materials. Their stiffness and tensile strength (even though only titanium and niobium carbide MXenes have been experimentally tested so far) exceed that of graphene oxide, molybdenum disulfide and all 2D oxides. Therefore, MXenes can be used to fabricate ultra-strong and lightweight structural composites. They have been applied as reinforcement materials to manufacture ultra-lightweight metal matrix nanocomposites based on aluminum, magnesium and lithium, increasing their specific yield strength up to ~60% without compromising the matrix alloy’s plasticity or resulting in a significant change in weight.
MXenes are also capable of sensing toxic gases and industrial chemicals with ultra-high sensitivity at room temperature because of their intercalation ability, diverse surface chemistry and charge transport properties. MXene epidermal sensors can help to monitor patients with certain types of diseases, while the sensing applications can expand further to ultra-thin membranes for sensing biomolecules such as DNA.
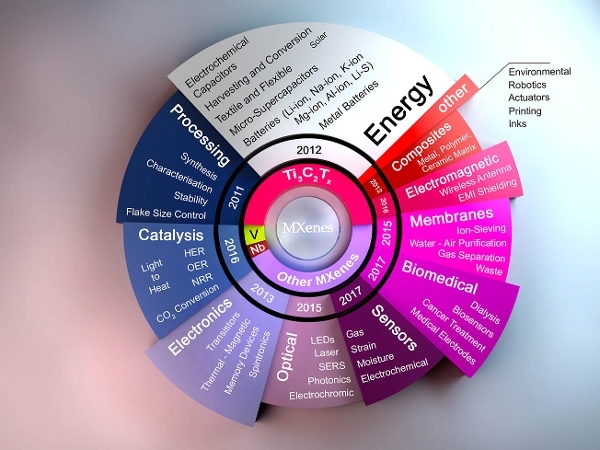
MXenes have shown remarkable performances in water desalination by osmosis and capacitive deionisation, turning brackish and seawater into drinking water. Other applications include photothermal cancer therapy using plasmonic properties, drug delivery using adsorption and intercalation, and dialysis, due to MXenes’ ability to adsorb urea – something that carbon sorbents cannot do.
Rapid growth
The potential of MXenes is continuing to expand at a tremendous pace with rep5orts on new compositions and structures, e.g. Mo₄VC₄, and a growing list of applications. Since the optical, electrical and chemical properties of these materials can be varied depending on the MXene type and composition, and can be finely tuned by changing the surface chemistry of forming solid solutions, the use of MXenes is expected to grow quickly.
In the past year, research has evolved and shifted towards new areas. In the next couple of years, we expect to see even more vibrant research on MXenes in applications such as water purification, metal alloys and composites, sensors and electronics. We also foresee that MXenes will continue to play a critical role in the development of next–generation energy harvesting, conversion and storage devices.