Lighting the way - fluorescent nanodiamonds in healthcare
Ben Miller, Erin Manning and Professor Rachel McKendry at University College London, UK, illuminate us on fluorescent nanodiamonds for early disease detection.
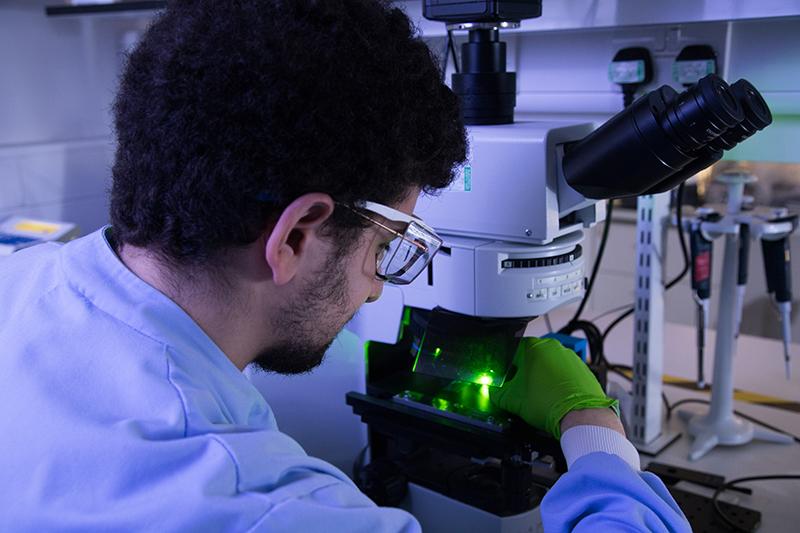
Since COVID-19 hit, many of us have now either used or are familiar with lateral flow tests. These low-cost, paper-based diagnostics have been widely available since the introduction of one-step pregnancy tests in 1988.
The porous structure of the nitrocellulose substrate (paper) conveys power-free fluid pumping – like water spreading through a paper towel – allowing multi-step diagnostics to be rapidly performed with little user input. Along with their low manufacturing cost, they are also suitable for testing in communities, in the home and other low-resource settings.
More recently, lateral flow testing has been applied to the diagnosis of infectious diseases, which has generated huge demand for cheap, rapid, accurate diagnostics. The pandemic has further highlighted the importance of analytical sensitivity – the smallest number of target biomarkers that the test can detect.
Detecting low levels of biomarkers is important when clinical levels are low and for early diagnosis, which is crucial in a pandemic to reduce onwards transmission and inform public health interventions.
Most current lateral flow tests are based on labelling biomarkers with gold nanoparticles, which are immobilised at the test line in the presence of the disease biomarkers. Gold nanoparticles absorb green light, producing the red line commonly seen on these types of tests to indicate a positive result. However, analytical sensitivity is limited by the absorption per particle, which can lead to low clinical sensitivity.
Under the spotlight
Fluorescent markers have the potential to be detected in much smaller numbers. Here, sensitivity is not limited by the absolute emission of the particles – as longer exposure times can be used – but the brightness relative to other sources of noise, such as from the sensor and background autofluorescence.
In the case of lateral flow tests, there is high, broad-spectrum, background autofluorescence from the nitrocellulose (paper) substrate, which masks lower signals resulting from smaller numbers of bound fluorophores, corresponding to lower analyte concentrations.
Many fluorescent labels have been used on lateral flow, such as organic fluorophores, quantum dots, europium nanoparticles and dye-encapsulating liposomes. Researchers have used varied approaches to circumvent the background autofluorescence, including upconverting phosphors to excite in the near-infrared region where the autofluorescence is lower; long-persistent phosphors to separate their signal by time-gating; or even reducing the fluorescence of the membrane itself.
In vitro diagnostic applications of fluorescent nanodiamonds build on previous work on in vivo imaging, which also faces the challenge of high background fluorescence.
Nanodiamond fluorescence originates from nitrogen-vacancy (NV) centres in the lattice. These NV centres comprise a substitutional nitrogen atom and adjacent vacancy (missing carbon atom), giving rise to an electronic structure that fluoresces red when pumped with green light. This electron arrangement also means that the fluorescence can be selectively modulated by an external electromagnetic field, allowing selective separation of their emission from background fluorescence.
In addition to their high brightness – by packing many NV centres into each nanodiamond – stability and easy functionalisation, this makes them ideally suited to sensitive, low-cost diagnostics.
In terms of the energy level structure, electrons in the triplet ground state (electron spin state ms = 0, ± 1) can be optically excited with green light, and then decay radiatively back to the ground state (conserving spin and emitting a photon). However, electrons with ms = ± 1 can also decay through a metastable dark state to the ground state with spin ms = 0 (not conserved), not emitting a photon. The 2.87GHz microwave field drives the ms = 0 electrons in the ground state to the ms = ± 1 levels, increasing the likelihood of decaying through the dark state, reducing the total fluorescence.
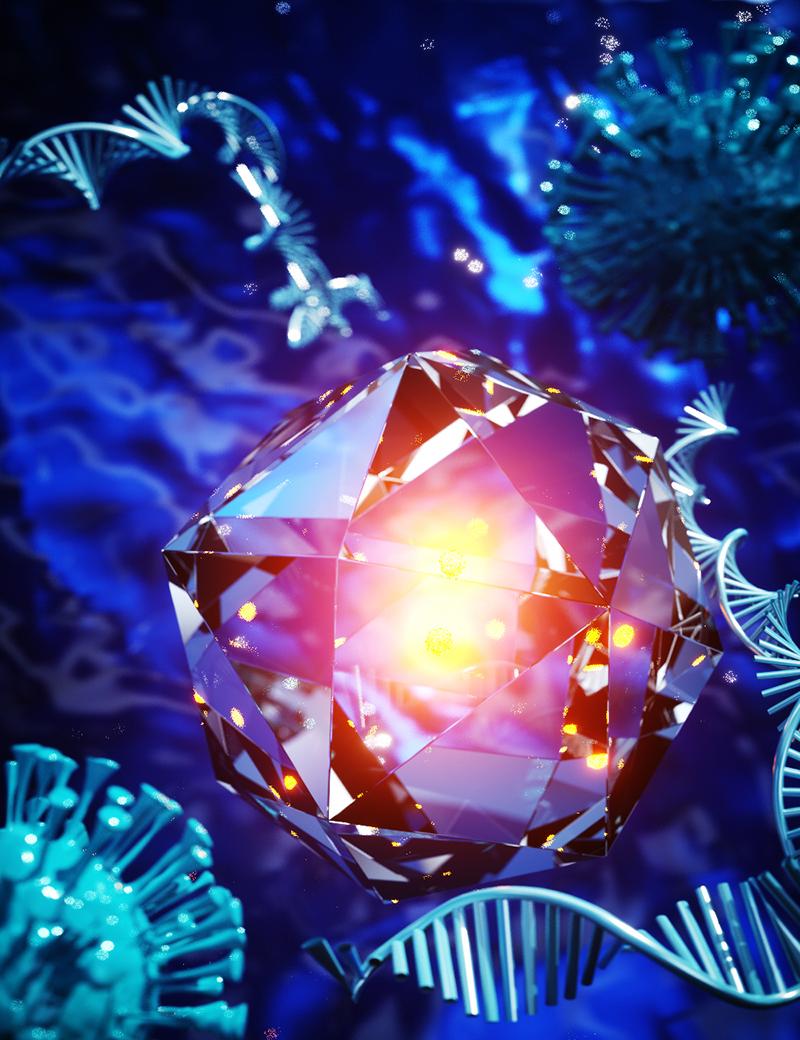
Nanodiamond discovery
Nanodiamonds were discovered by accident in 1963 by Soviet scientists. They found that nanodiamonds form from explosions of graphite-containing charges in detonation chambers.
Now, nanodiamonds can also be produced at low cost by milling or crushing of the material made by high-pressure, high-temperature (HPHT) synthesis, where a carbon source, such as graphite, is subjected to high temperature and pressure in press and precipitates around diamond seeds. Nitrogen impurities in the diamond lattice can then be transformed into fluorescent nitrogen-vacancy (NV) colour centres by irradiation and subsequent annealing.
Although, they were largely unused for many years after their discovery, today nanodiamonds are the subject of research for an enormous diversity of applications, from exploiting their semiconductor properties for diamond electronics, to energy storage, composite materials, and as quantum sensors for physical strain, temperature and electromagnetic fields. There are also numerous exciting medical applications, such as MRI contrast agents, drug delivery, in vivo imaging and sensing, and, most recently, as sensitive fluorescent labels for in vitro diagnostics.
Gold replacement
Nanodiamonds can be incorporated into lateral flow tests as fluorescent labels, in place of gold nanoparticles. Although nanodiamonds cannot be detected by eye, they can be imaged with a fluorescence microscope or lateral flow fluorescence reader.
The addition of a narrowband stripline resonator allows an amplitude-modulated microwave-frequency electromagnetic field to be applied, which causes the fluorescence emission from the nanodiamonds to modulate at the chosen frequency, while the autofluorescence from the background test strip is unaffected.
Taking a short series of images of the lateral flow test line and then applying frequency-domain analysis – equivalent to a Fourier Transform – makes it possible to separate the nanodiamond fluorescence from the background autofluorescence.
Recent work published in Nature shows that this leads to a 100-fold improvement in the lowest number of particles that can be detected over conventional fluorescence intensity imaging, giving a total 100,000 times more sensitive than gold nanoparticles, a detection limit of 0.5 particles per microlitre, or ~27 particles in total.
This fundamental limit was measured using an idealised assay with the binding of biotin and avidin – a very fast reaction. Extending this work to a more realistic assay for the detection of DNA amplicons gave a detection limit of 3.7fM or ~2,200 copies per microlitre, a 7,500-fold improvement over gold nanoparticles.
The difference comes down to non-specific binding, a universal challenge for biosensing. When measuring the smallest number of particles detectable, the negative control is a ‘true blank’ with no nanodiamonds, so the signal measured is just background noise. However, in the sandwich assay, the negative controls contain nanodiamonds, some of which bind non-specifically at the test line, giving a small signal that obscures lower concentrations, reducing the improvement over gold nanoparticles.
Nonetheless, this is a significant improvement, and, with the addition of a short 10-minute isothermal amplification step, it allows single-copy detection of HIV RNA.
Showing sensitivity
This greater analytical sensitivity means that lower biomarker concentrations can be detected, allowing tests using fluorescent nanodiamonds to be more broadly applied to detect diseases with lower clinical levels, or at an earlier stage, which could reduce the risk of transmission, and improve prognosis with earlier treatment. However, significant challenges remain before adoption into real diagnostic tests is possible.
The key drawback is that a fluorescence reader is required for readout, as is the case with all fluorescent labelling, while gold nanoparticle-based lateral flow assays can be read by eye or simply with a smartphone camera.
Although any fluorescence reader can be used to perform the modulation (giving the additional 100-fold sensitivity improvement), the reader must also be capable of applying a microwave-frequency electromagnetic field, even if the required components are small and inexpensive. The use of a reader also conveys the advantage of inherent data capture, allowing transmission to centralised healthcare systems and reducing the risk of losing patients to follow-up care.
Low-cost, portable, re-usable fluorescence readers have been demonstrated for lateral flow tests. Significant research has been undertaken on using smartphone cameras for fluorescence imaging. Their increasing adoption allows for a reduction in cost using the camera and optics already built in, and powering from the internal battery.
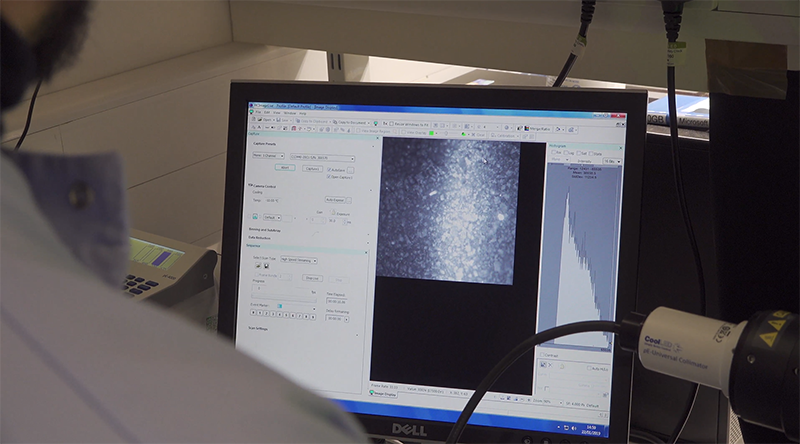
In addition to reader development, there are also remaining challenges around adapting nucleic acid detection assays to low-resource settings. In particular, extraction and purification often require time and a laboratory centrifuge, although there are reports of point-of-care RNA extraction. For this reason, nanodiamonds might find quicker adoption in antigen (or rapid) testing, where lateral flow technology is already widely used with other nanoparticles, such as HIV and COVID-19 antigen testing.
Nanodiamond-based lateral flow assays for detection of HIV capsid protein and Ebola glycoprotein have been published. Interestingly, the detection limit improvements conveyed might also be more impactful in an antigen test, where they translate directly into an improvement in analytical sensitivity, as opposed to amplification-based tests, where a longer amplification step, generating more amplicons, allows for the use of less sensitive nanoparticles at the expense of longer test times. The same is true for direct detection of nucleic acids with no amplification step.
Assay sensitivity will also be dependent on the binding kinetics of the disease biomarker to the recognition molecules, and the ability to reduce non-specific binding. These challenges are present in all nanoparticle-based diagnostic development, and are crucial to overcome to realise the full potential of nanodiamond-based diagnostics.
Nanodiamonds are broadly applicable to a range of nanoparticle-based diagnostic assay formats by simply switching out the existing nanoparticles, thanks to their ease-of-functionalisation and stability. They are also target-agnostic, suitable for communicable and non-communicable diseases as well as other biomarkers, drug, veterinary and environmental monitoring.
Further work is needed to identify the areas where the strengths of nanodiamonds can be most impactful, but this technology provides an exciting direction for the future of low-cost diagnostics. Sensitivity is a key barrier to their widespread adoption, which could see a fundamental shift in healthcare provision.