Lessons from the lithosphere
Dr Daniel Fernández-González and Dr Luis Felipe Verdeja AIMMM, from the University of Oviedo, Spain, reflect on the distribution of elements in the Earth’s crust and their order in nature and the environment.
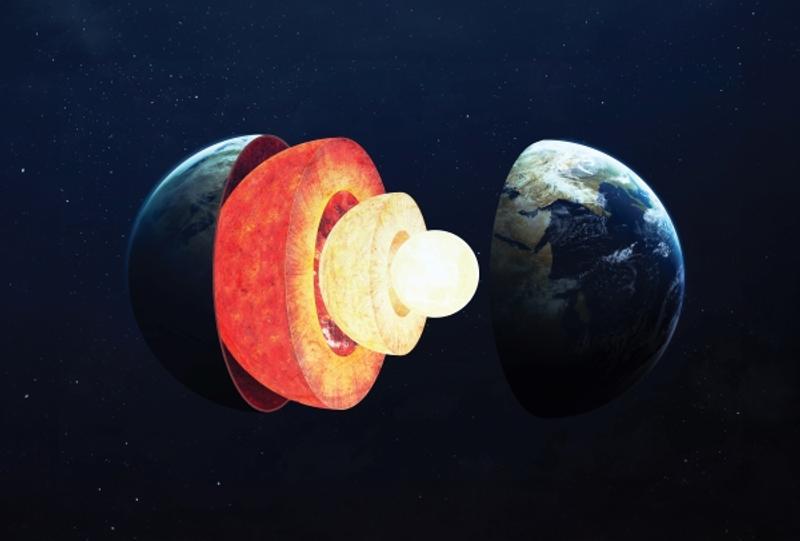
Chemical elements that constitute the lithosphere are not those that humans would desire, and they are probably not in the place where they would have been preferred, but they appear as and where they are as a result of a “superior entity”, as specified by eminent geochemist William Fyfe in Geochemistry.
The list of the most abundant elements in the Earth’s crust starts with oxygen, silicon, aluminium and iron, with alkali earth elements (calcium and magnesium) and alkali elements (sodium and potassium) further down.
The ninth most abundant is titanium (0.63%), which is the first element that is not in a concentration of >1.00%. Sulphur and carbon are 16th and 17th on the list, with average concentrations of 340ppm and 180ppm, respectively. The atomic weight of sulphur is almost three times greater than that of the carbon.
Finally, another important element is hydrogen, which is 10th with 1,250ppm, forming hydrated compounds in the lithosphere due to its low atomic weight.
These values of the average concentration of the elements in the Earth’s crust explain why the most used metallic materials are those based on iron and aluminium, why silicon is an important element in the manufacture of electronic devices and photovoltaic cells, and why aluminum is the most used element for carrying electricity.
It also explains why the most common material for structural applications is concrete reinforced with steel fibres.
The distribution of elements in the Earth’s crust leads to two concepts – basic materials and critical materials.
Basic materials consist of the following properties:
- The raw materials used in its manufacture are abundant and are one of the 10 most abundant elements in the lithosphere.
- The energy required to obtain the final product is not significantly high enough to involve a final competitive price for the product.
- The possibilities of recycling or reuse of the final product once the lifecycle ends are high. In the same way, the by-product generated in the extraction either can be used in other processes or products, or does not involve an environmental risk during its storage in controlled dumps (or spoil tips).
Critical materials have the following characteristics:
- The elements required for the finished product are not the most abundant in the Earth’s crust. Their concentration is not high – i.e. it does not exceed the tens of ppm – such as for cobalt, niobium, lithium, samarium, tantalum or germanium.
- The energy required to obtain the element or pure compound is much higher than that required in the case of basic materials.
- Residues and by-products generated in the extractive process are usually difficult to recycle and store.
Basic materials can promote development in poorer countries, the growth of emerging ones and the sustainability of developed economies. On the other hand, critical materials, which are demanded by society for both structural and functional applications, are produced in quantities that barely exceed millions of tonnes. Compare this to basic materials, which are produced in quantities that exceed 1Mt or even 1,000Mt (steel and concrete).
When considering materials selection, all these factors must be taken into account.
Empathising with entropy
An element or compound is extracted from the oxidised, sulphurated or carbonated raw materials.
The laws of thermodynamics dictate that all processes, spontaneous or irreversible, are associated with an increase in the entropy of a system. The thermodynamic equilibrium is a consequence of a variation of entropy, and any material in the absolute zero of temperatures (i.e. 0K or -273°C) has perfect order.
The spontaneous processes involved in extraction result in an increase of entropy due to the release of gases, mainly CO₂, and to a lesser extent SO₂, which are compounds of high entropy. Also, the production of residues, which are also disordered materials with levels of entropy comparable or greater than that of the starting raw materials.
As we know, oxygen is the most abundant element in the lithosphere, and the thermodynamics certify that the most stable compounds (and therefore of lowest entropy) are oxidised compounds.
It is also possible to find stable metal sulphides, metal silicates and metal carbonates. However, the possible interactions of these compounds with the environment (particularly water) does not lead to soluble compounds – something that could dramatically increase the entropy.
It could be argued, on the other hand, that reduced solubility means interaction of the minerals-rocks with the environment does not lead to noticeable increases of the Earth’s disorder – something naturalists aspire towards.
Nevertheless, human intervention is necessary. Take the maintenance of forests, for example, this provides significant benefits in reducing fire risks. This ties in with the metaphysical hypothesis that humans are tasked with the correct administrative care of the Earth.
Recovering ground
Lost entropy in the residues can be recovered by thinking of residue as an abandoned product and, consequently, susceptible to being transformed into ordered elements-materials of low entropy.
Correct management of waste can restore entropy. If raw minerals with a level of entropy are considered as the starting point, it would be possible to decipher that the residue would have a level of entropy similar to that of the starting raw material, and that the environmental interaction of the residue would not create compounds with high solubility.
Concentrated solar energy is an alternative that could help obtain stable compounds in water from residues that are thermodynamically unstable, or to obtain a new compound of high thermodynamic stability and low entropy that would be susceptible to being recycled or stored with the suitable environmental security.
It is possible, however, that, after the classical extractive metallurgy treatments, or after the most novel processes with solar energy, a product is obtained that is not suitable for reuse. This would be a scenario where the volume of residue does not reach the minimum amount to be commercially exploited.
It is common in this situation for the current managers of the controlled dumps (or spoil tips) to store the by-product in such a manner that it could be reused in the future if the conditions change, or the accumulated quantity of by-product is later sufficient to be commercially attractive.
For this purpose, management of residues for by-products should ensure that the quality and the quantity stored is sufficiently documented for future operations. This operative protocol would also contribute to reducing the entropy of spontaneous processes.
Other avenues for reducing entropy is recovery of entropy liberated during the release of gases in the atmosphere. This could be achieved by stabilisation of CO₂ by means of a cellulose polymer – (C₆H₁₀O₅)n·H₂O – through the carbon hydrate produced by plants, the mineralisation of CO₂ and SO₂ as chemical compounds in rocks or slags, and the mineralisation of nitrogen oxides, benzo(a)pyrenes, dioxins and other compounds resulting from the bonding of aromatic compounds.
In factory production of coke, the most dangerous environmental organic compounds are collected and treated in auxiliary installations to the coke ovens and separated from the lightest products (aliphatic-aromatic compounds derived from the benzene) to the heaviest products (pitch and tar). Future technology can provide improvements in efficiency.
Electrical power generation through the combustion of coal produces carbon dioxide emissions, SO₂, NOx and organic macromolecules – generated as a result of the condensation of the benzene rings – all more detrimental for the environment than the CO₂ molecule.
Therefore, it is necessary to take into account that 200ppm of CO₂ in the atmosphere are necessary for the plants, although the CO₂ concentration has changed from 315.98ppm in 1959 to 414.89ppm in 2022.
Finally, it is also important to consider that the CO₂ released during the calcination of a carbonate is not the same as the CO₂ produced in the oxidation of a combustible. Therefore, in the calcination of the carbonate, the CO₂ would be free of organic molecules if renewable energies are used for calcination.
Consequently, it would be possible to contribute to a decrease in entropy growth generated by the spontaneous processes if the quick lime necessary for the mineralisation of both the CO₂ and the SO₂ could come from the calcination of carbonates by means of concentrated solar energy.