A hard tablet to crack – making pills
Materials science challenges in manufacturing everyday medication.
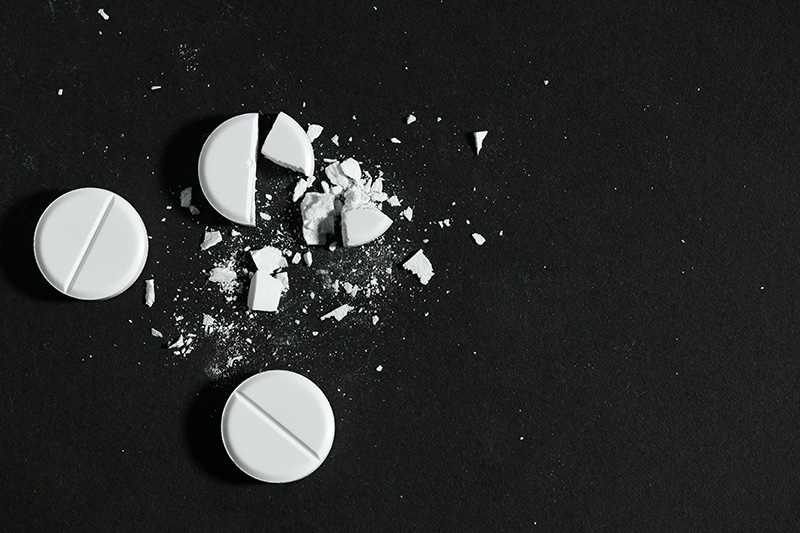
Counting aspirin alone, the world consumes over 58 billion tablets per year, according to a Washington Post article on Aspirin: the worlds most popular pill turns 100.
If the bioavailability of the drug permits, pills are also by far the most convenient form of drug dosage. You can take tablets anywhere, administer them whenever you want, and you do not have to worry about specialised storage conditions.
Mass production is also relatively easy and drug dosing can be controlled precisely, easing the regulatory process. So, it comes as no surprise that pill manufacturing remains a major topic of interest in the pharmaceutical sciences.
But the unfortunate reality is that tablets can fail. During the production process, they can chip at their corners (chipping), split in two (lamination), or the top part of a rounded tablet can come off (capping). If not corrected early in production, this can make many of the products unusable as the speed at which a tablet dissolves, and thus the rate of drug release, becomes unpredictable, and coating of the tablets becomes problematic. This is unacceptable when many drugs require a slow and steady release profile, or a protective coating against light or stomach acid.
Even without these issues, a lack of control over quality can delay clinical trials, thereby lengthening the time it takes for new drugs to reach the patient. Defects are therefore highly undesirable but also immensely complex to manage.
Pressing a powder
All the starting materials are powders. These are granular materials and cannot simply be considered either solids or liquids. Each individual grain has its own particular shape, size and even mechanical properties. These grains can all push, slide and break against each other.
Moreover, pharmaceutical powders are often much softer than, for example, sand, meaning they can deform elastically or plastically as well.
The reality is that no tablet is made from a pure drug powder. Drugs are frequently crystalline and end up making very poor tablets by themselves, either being too insoluble or just falling apart. Other ingredients are added to the powder mixture to help the tablet stick together (binders) or help the drug dissolve more easily (disintegrants).
Common binders vary but are all polymeric in nature. Some are excipients – i.e. ingredients alongside the active ones in medication. Examples include natural polymers (gelatin, starch, lactose), semi-synthetic polymers (sodium carboxymethyl cellulose, hydroxypropyl cellulose), microcrystalline cellulose, polyethylene glycol and carbomers.
Natural disintegrants are similar, with such examples as alginic acid, powdered cellulose, guargalactomannan and again microcrystalline cellulose.
In general, there is no fixed set of powders or types of powder that have to be in a formulation. For example, not all tablets may need a disintegrant. Excipients are powders that might help alleviate some of the problems that are encountered during the formulation process.
However, as you might expect, adding a disintegrant to the formulation also changes the mechanical properties of the tablet, possibly weakening it, causing you to need more binder again. This is exactly the formulation problem, where many different necessary components together interact to give the final properties.
Conversely, the amount of active pharmaceutical ingredient (API) needed for an effective dose can also be very low. In these cases a tablet made out of pure API would be too small to produce well, and it would be harder to control the dosing and quality.
Similarly, tiny tablets might dissolve too fast. In that case, you want to combine the API with an excipient that adds volume but is less soluble (but not too poorly soluble) to decrease the dissolution rate. In general, pharmaceutical scientists try to go for a set tablet volume or mass (say 400mg) and try to change the composition within these requirements.
To make a tablet
A feeder deposits a controlled amount of powder into a cylindrical metal cavity, known as the die. The powder is then compressed by two metal punches, one at each end of the die. When the required density has been reached, the punches release the pressure and move apart again. The newly formed tablet is finally pushed out of the die by the lower punch.
However, this deceptively simple-sounding process quickly becomes incredibly complex due to the nature of the starting materials.
With all of our current-day knowledge, we still cannot say we fully understand the behaviour of sand, let alone soft pharmaceutical powders. For example, compressing a failing tablet to a higher density to make it harder will not necessarily work. Some defects actually become more frequent for denser tablets, and a denser tablet may no longer dissolve. As a consequence, tablets can end up breaking when a different pharmaceutical powder is used, or the production speed or pressure is changed.
Modelling behaviour
The powders’ complexity therefore eludes any straightforward analytical description of tablet formation. Numerical models have become a common method for tackling problems in pill production. In particular, the finite element method (FEM) has become a popular tool, with a good track record of indicating potential defects as well as final density.
In FEM, the total object is divided into many small volumes of homogeneous material. At the heart of these methods are constitutive models that describe the mechanical behaviour of that very small volume of powder – for example, its stiffness and yielding strength. In tablet compaction, the density-dependent Drucker-Prager Cap (dDPC) model is a common choice.
Combining FEM and dDPC enables computer simulations of the powder compaction process to investigate variations in stress and density within the pill during each stage of the process.
The stresses that arise are directly related to defect occurrence, meaning, for a given powder, it is possible to see how variations in the tablet’s density and shape can change the chance of defects.
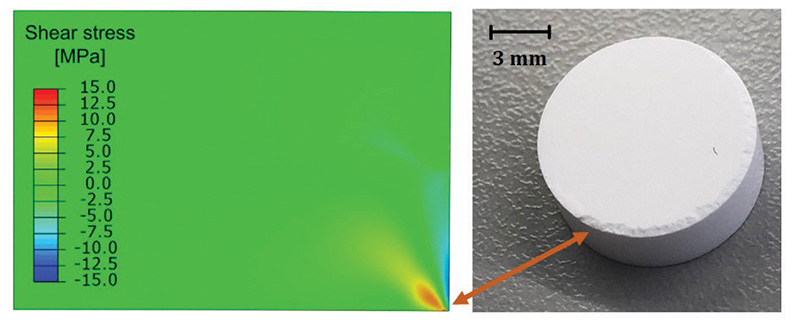
By far the trickiest part of this process is calibrating the dDPC model. Pushing, sliding, breakage and deformation of the particles make powder behaviour nearly impossible to predict. Taking several tens of grammes of powder and making tablets at different densities (around 60 or so) is important to provide data that help determine the model’s parameters and carry out simulations.
During calibration, the model lumps together all complex interactions to give one overall mechanical response. This eases things but the model is specific to the powder used.
And while compaction pressure and pill shape can be optimised once the powder is known, the powder is usually unknown. It is therefore not only the density and shape of the tablets that varies, but also the starting powders’ composition. This introduces us to the dimensionality curse within the formulation problem.
The formulation problem
Suddenly, we must evaluate thousands of different powders, as even changing the concentration of a single component can change the final tablet’s mechanical or dissolution properties. Too many options – that is the dimensionality curse.
To pick a combination of powder composition and tablet shape/density that meets all the pharmaceutical requirements – bioavailability, dissolution, degradation resistance, etc. – but also create a perfect tablet becomes challenging to say the least.
So, even with computer simulations for testing the tablet shapes and densities, we must still make about 60 tablets per powder formulation. Carrying out all of these experiments requires a considerable quantity of drug that is often scarce in the early stages of development, but also can be time consuming. In the pharmaceutical industry, an extra month of development can quickly cost millions of pounds that could otherwise have been invested in other activities.
The scarcity of data similarly precludes the use of most statistical and machine learning methods. Together, these challenges create a strong drive for physics-based modelling of powder formulation and direct compression.
Powdering up
Several years ago, Professor James Elliott FIMMM of the University of Cambridge, UK, and Dr Ioannis Fragkopoulos of pharmaceutical company Novo Nordisk A/S, Denmark, set up a joint project on modelling tablet compaction. This started off with building typical simulations that combine FEM and the dDPC model, to replicate results previously reported in the literature.
When looking at how the experiments are used to inform the simulations, most engineers apply a trial-and-error approach. Although effective in the end, this means that every time a new formulation is tested, the experiments are repeated to parametrise the simulations. This partly obviates the reason for doing simulations in the first place – to save time and material.
Tackling this problem requires expertise in scientific computing – a discipline lying between physics and computer science. It can find out why using a different set of parameters can suddenly predict an unrealistic explosion of a tablet.
A deeper understanding is also needed of how the experiment (or reality) is related to the dDPC model. By understanding how theory relates to reality, and also how it doesn’t, we make sure that the simulations become as meaningful and predictive as possible. Eventually, this combination of physics, computer science and mathematics gives us a rigid set of rules by which to determine the set of model parameters that best describe reality.
A year after starting the project, these rules have been codified and an automated workflow created that, given experimental data, is guaranteed to create a good set of simulation parameters.
The gap between finishing experiments and obtaining the parameters used to be hours, but is now just the push of a button away. Also, the workflow is so stable that 30 tablets are needed per powder instead of 60. At worst, this saves us a third of the time and half the powder. Nonetheless, the formulation problem is still lurking – 20% of 1,000 combinations is still too much.
Going against the grain
An interesting observation along the way can sometimes become the key to solving a later problem. If a die is filled with two powders – one horizontal layer of powder A and one of powder B – and they are compressed, we find that it behaves strikingly similar to compressing a perfect mixture of powder A and B.
So, what if this also works for a small volume of powder? Say, the amount we are describing with the dDPC model? If so, we can predict the parameters of new formulations by combining the parameters of individual component powders. We could therefore create simulations of formulations that have not been made yet.
The principle is more generally referred to as a set of mixing rules and is the basis for a new virtual formulation tester.
It works as follows – for every component that may be part of a new formulation, we do the experiments and use the workflow to generate the model parameters. Then, if a new formulation needs to be tested, we can skip doing the experiments and determine the simulation parameters using the mixing rules instead.
Finally, the simulations are executed and inform us how the new formulation will behave when compressed in different ways – varying shape, density and pressure. The virtual tester can therefore tell us if a tablet made with this new formulation might develop a defect. Testing combinations virtually can therefore help pick the most promising formulations for further physical experimentation.
Although virtual testing is a great help in reducing the need for experiments, and wasting precious powders and time, it does not completely circumvent the curse of dimensionality. Simulations take time as well, and we may still not be able to evaluate all possibilities. However, our reach has extended significantly, and may do so even further when virtual testing is combined with design of experiment principles.
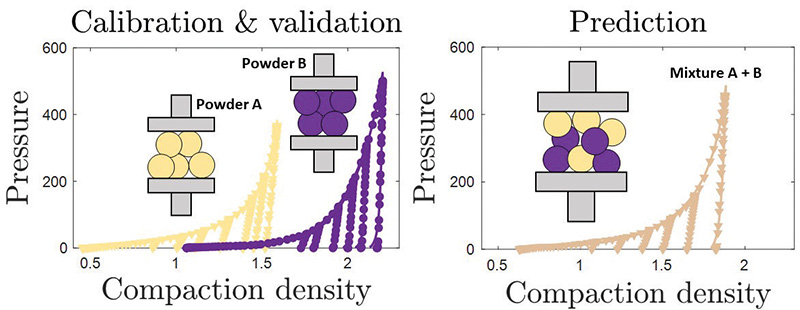
Reformulating the future
New physics-based methods are on the rise for predicting the path from powder to tablet. Despite growing interest in machine learning, with some computer help, physics still has much to offer.
New methods can be calibrated using only a few powders, but can predict the behaviour of many mixtures forming tablets at various densities, pressures and shapes. This is useful within pharmaceuticals and can also be applied to detergent, ceramic and metal powders.
Challenges naturally remain. Some phenomena fall outside of the current models. Tablets occasionally stick to one of the punches, and different lubrication methods reducing the friction between the tablet and the tooling are not yet included.
Limitations may also appear if the powders’ grains are too different in size. But facing these challenges leads to new insights. In this case, working on the mixing rules revealed that having a softer powder on the tablet outside may reduce defects.
A common challenge is that the pure component powders do not always form a cohesive tablet, meaning parameters cannot be obtained for these powders. This has now been solved, at least partially, and we can estimate parameters of pure powders that do not make tablets, from a few mixtures that do contain the powder. This study is under review and due to be published in Advanced Powder Technology.
The biggest problem now is that we do not know how the compression speed affects the model parameters.
Current concerns regarding the safety of titanium dioxide (TiO2), a common component in tablet coatings, had the EU considering restrictions to its usage. Moving away from TiO2 coatings may require the reformulation of 90% of all tablets.
With the new tools, we may ease these potential transitions and create the powder formulations of the future. However, it goes without saying that what we have now is not perfect. Granular matter is still too complex to completely comprehend. To predict fully what a powder does, that will probably take us a lifetime.