3D-printed superalloys creep through heat to reduce defects
A heat treatment that transforms the microscopic structure of 3D-printed metals makes the material stronger and more resilient in extreme thermal environments, according to researchers at the Massachusetts Institute of Technology (MIT), USA.
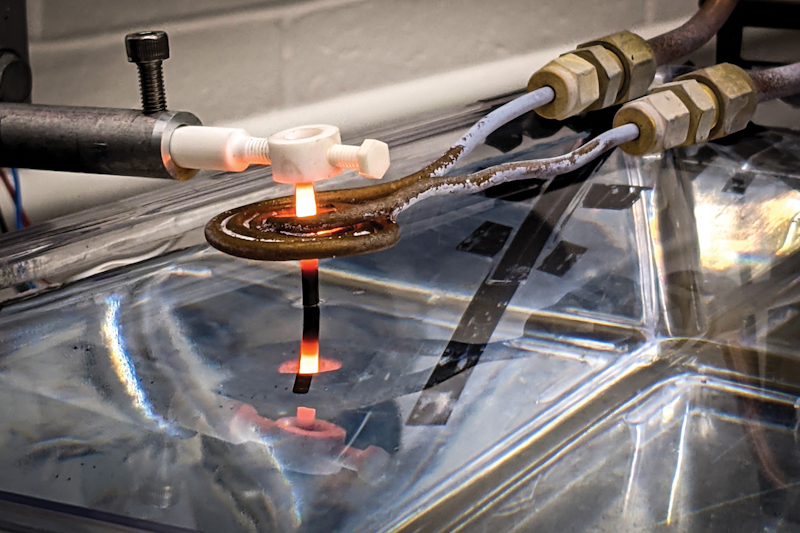
They suggest this method could lead to 3D-printed high-performance blades and vanes for power-generating gas turbines and jet engines, with new designs and improved fuel consumption.
Gas turbine blades are currently manufactured by conventional casting and made from heat-resistant metal alloys, due to the very high-speed rotation in super-hot gas that is required to generate electricity in power plants and thrust in jet engines.
Hitherto, 3D-printed metals have been subject to creep and permanent deformation. According to the MIT team, past research has found that printing metals produces fine grains, which are tens to hundreds of micrometres big.
Zachary Cordero, the Boeing Career Development Professor in Aeronautics and Astronautics at MIT, says that this would result in a gas turbine having a shorter life or being less fuel efficient.
Through additional heat treatment, the materials fine grains are transformed into larger ‘columnar’ grains to create a sturdier microstructure that minimises creep, as the columns are aligned with the axis of greatest stress.
The research group calls this a form of ‘directional recrystallisation’ – where a material is passed through a hot zone at a precisely controlled speed to meld many microscopic grains into large and more uniform crystals.
Invented more than 80 years ago and applied to wrought materials, the MIT group has adapted it for a 3D-printed nickel-based superalloy, which is commonly used in the hot section of a gas turbine engine – specifically IN738LC.
3D-printed samples of rod-shaped superalloys are placed in a room-temperature water bath below an induction coil. Each rod is slowly drawn out of the water and through the coil at various speeds and heated up to 1,200-1,245°C – the range between solvus and solidus where expected recrystallisation sets in.
By drawing the rods at 2.5mm/h at 1,235°C, they are able to create a steep thermal gradient that triggers microstructure.
Cordero explains that the defects called dislocations annihilate and reconfigure when heated and the grains are able to grow. After cooling, optical and electron microscopy is used to examine the microstructure.
The team claims to have demonstrated that manipulating the draw speed and temperature tailors the growing grains, creating regions of specific grain size and orientation. Cordero says that this control can enable manufacturers to print blades with site-specific microstructures that are resilient to certain operating conditions.
'[A draw rate of] 2.5mm/h is acceptable for smaller components and for larger, high-value components where creep-resistance is essential. We are developing ways to accelerate the process so it can be applied more generally,' he continues.
'The diffusional creep rate scales as 1/D3 where D is grain size. Conventionally recrystallised materials have grain sizes of 100µm. We see grains as large as 1cm. Hence, we expect creep rates 10⁶ slower in our materials as compared to conventionally recrystallised materials. At the anticipated creep rates, additively manufactured nickel-base superalloy blades and vanes could feasibly be used in gas turbine applications.'
They now plan to test the heat treatment on 3D-printed geometries that more closely resemble turbine blades as well as examine the heat-treated structure’s creep resistance.